What is the difference between steric and torsional strain?
While both types of strain can be minimized by rotating the molecule around a bond, steric strain is not always minimized by this process. This is because steric strain is due to the close proximity of atoms, and this proximity can be difficult to change by rotation alone. Torsional strain, however, can be reduced by rotating the molecule around a bond to align the atoms in a way that minimizes the repulsion between their electron clouds.
Let’s dive a little deeper. Imagine a molecule with two bulky groups attached to a single bond. As these groups rotate around the bond, they can either be positioned close together (causing steric strain) or further apart. Steric strain can be minimized by rotating the groups away from each other, but only to a certain extent. If the groups are very bulky, there may be no orientation where they can be positioned without experiencing some steric strain.
Torsional strain is a bit more straightforward. It arises from the repulsion between electron clouds on atoms that are bonded together. Think of it like the repulsion between two magnets with the same poles facing each other. The further apart the magnets are, the less repulsion they experience. Similarly, the further apart the electron clouds are, the less torsional strain the molecule experiences. By rotating the molecule around a bond, you can adjust the alignment of the electron clouds to minimize this repulsion and reduce torsional strain.
In summary, steric strain is caused by the close proximity of atoms, while torsional strain is caused by the repulsion of electron clouds. Both types of strain can be minimized by rotating the molecule around a bond, but steric strain can be more difficult to minimize than torsional strain.
What is torsional strain?
Let me explain. Imagine a molecule as a series of connected sticks. Each stick represents a chemical bond between atoms. If you rotate one stick relative to the others, you might encounter resistance. This resistance is torsional strain. It arises from the repulsion between electron clouds of atoms that are close together.
Think of it like this: If you try to rotate a propeller on an airplane, you’ll feel resistance because the blades are trying to move through the air. The same principle applies to molecules. The atoms are trying to move through each other’s electron clouds, and this creates a strain.
The staggered conformation is like having the propeller blades set at an angle to each other, minimizing the amount of air resistance. The eclipsed conformation is like having the propeller blades all lined up, maximizing the amount of air resistance.
In a staggered conformation, the atoms are positioned so that they are as far apart as possible, reducing electron cloud interactions. In an eclipsed conformation, the atoms are directly in front of each other, leading to a higher degree of electron cloud repulsion and thus, greater torsional strain.
For example, in ethane, the staggered conformation is more stable than the eclipsed conformation because the hydrogen atoms are further apart in the staggered conformation. This difference in stability is what gives rise to the torsional strain.
Torsional strain is an important factor in determining the conformation of molecules and can have a significant impact on the molecule’s properties. Understanding torsional strain can help you predict the shape and reactivity of molecules.
What is a steric strain?
We’ve all been there: crammed into a small space with others, feeling uncomfortable and wanting to move away. Molecules experience something similar called steric strain, which happens when atoms or groups of atoms are squeezed too close together.
Think of it like this: atoms are like tiny balls with a cloud of electrons surrounding them. These electrons repel each other, kind of like magnets with the same poles facing each other. When atoms get too close, their electron clouds push against each other, creating a kind of “stress” within the molecule. This is steric strain.
Steric strain can affect a molecule’s shape, stability, and reactivity. Imagine trying to fit a large object into a small space. It’s going to be difficult and might even be impossible. The same applies to molecules. If atoms are forced into a tight space, it can make the molecule less stable and more likely to react in unexpected ways.
There are a few things that can contribute to steric strain:
The size of the atoms or groups of atoms: Larger atoms or groups of atoms are more likely to clash, leading to greater steric strain.
The arrangement of the atoms in space: Some molecular shapes are more prone to steric strain than others. For example, a molecule with a lot of bulky groups crowded together is likely to experience more steric strain than a molecule with the same groups spread out.
The distance between the atoms: Obviously, the closer the atoms are, the more likely they are to experience steric strain.
Let’s look at a simple example. Imagine a molecule with two large groups of atoms attached to a central carbon atom. If these groups are bulky and close together, they will experience steric strain because their electron clouds will repel each other. This can make the molecule less stable and more reactive.
Steric strain is a fundamental concept in organic chemistry, and it helps us understand how molecules behave and react. So, the next time you’re feeling cramped, remember that molecules experience a similar discomfort called steric strain.
What is the difference between steric strain and gauche?
Gauche conformations occur when two bulky groups, like methyl groups, are positioned at a 60-degree angle to each other along a carbon-carbon bond. This arrangement leads to steric strain, which is the repulsion that arises when these groups get too close together.
Imagine two people trying to squeeze through a narrow doorway. They’ll bump into each other, creating a tense situation, similar to the interaction of those bulky groups in a gauche conformation.
The anti conformation, on the other hand, represents a more relaxed state where the bulky groups are positioned 180 degrees apart. In this arrangement, the groups are far enough apart to minimize the repulsive forces.
Think of it like having two people walking in opposite directions on a wide street. They have ample space, and there’s no need for bumping or discomfort. This analogy highlights the key difference between gauche and anti conformations: gauche conformations are associated with higher energy levels due to steric strain, while anti conformations are lower energy and more stable due to the minimized interaction between bulky groups.
Steric strain is a crucial factor in understanding the relative stability of different conformations of molecules. It arises from the repulsive forces between electron clouds of atoms or groups that are forced into close proximity. This repulsive force pushes the molecule towards a more stable conformation, often by minimizing the angle between bulky groups.
Gauche conformations, while present, are generally less favored due to the steric strain they experience. This concept of steric effects plays a critical role in determining the shape and reactivity of molecules.
Does steric strain increase stability?
Let’s break this down a bit further. Steric hindrance is all about the physical space that molecules occupy. When groups get too close, their electron clouds start to bump into each other. This creates a kind of “pushing” force, making the molecule less likely to exist in that configuration.
Here’s a real-world analogy. Imagine you’re trying to fit a bunch of bulky furniture into a small room. The larger the furniture, the harder it is to fit everything in comfortably, and the more likely you are to bump into things. Similarly, bulky groups in a molecule make it harder for them to bond effectively, and they might even prevent certain reactions from happening.
For example, imagine you have two molecules trying to bond together. If one of the molecules has a very large group attached to it, that large group will create steric hindrance and make it difficult for the two molecules to get close enough to form a stable bond.
In essence, steric hindrance is a bit like a molecular traffic jam. The more crowded the space, the more difficult it is for molecules to move around and interact effectively. This leads to a decrease in stability, because the molecule is in a higher energy state.
Is Van der Waals strain the same as steric strain?
Steric strain, also known as van der Waals strain, happens when atoms or groups within a molecule are forced too close together. Imagine these atoms like tiny balls trying to fit into a small box. If they are squeezed too tight, they bump into each other, increasing the molecule’s energy. This increase in energy is what we call steric strain.
The key to understanding steric strain lies in the van der Waals radii of atoms. Every atom has a certain radius, which defines the space it occupies. When atoms approach each other, they experience a repulsive force if they get closer than the sum of their van der Waals radii. This repulsive force is the origin of van der Waals strain.
Think of it like this: Imagine you have two inflatable beach balls. If you push them close together, they will resist, creating pressure. This pressure is similar to the van der Waals strain experienced by atoms in a molecule. The closer they are forced to be, the greater the strain.
Steric strain is important because it impacts a molecule’s shape and stability. When there is significant steric strain, a molecule might adopt an unusual shape to minimize the repulsive interactions between its atoms. This can influence the molecule’s reactivity and its ability to interact with other molecules.
Steric strain plays a crucial role in various chemical processes, including:
Organic reactions: Steric strain can affect the rate and outcome of chemical reactions.
Protein folding: Steric strain influences the way proteins fold into their specific three-dimensional structures.
Drug design: Understanding steric strain is essential for designing drugs that fit into their target proteins effectively.
By understanding steric strain, we can better predict the behavior of molecules and design new materials with desired properties.
What is torsional vs angular strain?
If you look at cyclopropane from the perspective of one of its carbon-carbon bonds, you’ll notice that the hydrogen atoms are all in an eclipsed conformation. This means that the hydrogen atoms are directly opposite each other, creating a significant amount of torsional strain.
Think of it like trying to force two people to sit directly across from each other in a small, cramped car. It’s uncomfortable and creates tension!
Torsional strain is the energy penalty associated with this kind of eclipsing interaction, where electrons in the bonds of neighboring atoms repel each other.
But that’s not all! Cyclopropane also experiences angular strain, also known as ring strain. This is because the carbon atoms in a cyclopropane ring are forced to form bond angles of only 60 degrees. Ideally, these angles should be closer to 109.5 degrees, which is the preferred angle for a tetrahedral carbon.
This deviation from the ideal bond angle causes a lot of stress on the molecule, leading to angular strain.
Imagine trying to squeeze a balloon into a small box. You’re forcing it into a shape it doesn’t want to be in, and it creates tension and pressure.
In addition to the torsional and angular strains, cyclopropane also faces steric strain due to the close proximity of the atoms, causing repulsion between their electron clouds.
So, to summarize, cyclopropane is a highly strained molecule due to the combined effects of torsional strain, angular strain, and steric strain. It’s like a tiny molecule under a lot of pressure, constantly trying to find a more relaxed state.
Which has more torsional strain?
Think of it like this: Imagine you’re trying to cram two large boxes into a small closet. You’ll have to force them in, and it’ll be a lot of effort to get them to fit. That’s similar to what happens with the eclipsed conformation. The electron clouds of the substituents are trying to occupy the same space, which leads to a lot of repulsion and energy.
On the other hand, the staggered form is like having the boxes arranged in a way where they don’t touch each other. This is a much more comfortable and stable arrangement, just like the staggered conformation of a molecule. In the staggered form, the electron clouds are further apart, which reduces electron-electron repulsion and results in lower energy and less torsional strain.
Remember, the eclipsed conformation has the maximum torsional strain, and the staggered conformation has the minimum. This is a key concept in understanding the conformations of molecules and how they behave.
Is angle strain and torsional strain same?
Angle strain occurs when bond angles in a molecule deviate from their ideal values. This deviation can happen when atoms are forced to adopt a geometry that is less stable than the most favorable arrangement. For instance, in a cyclopropane molecule, the carbon atoms are forced into a triangular shape with bond angles of 60 degrees, instead of the more stable tetrahedral angle of 109.5 degrees. This distortion leads to an increase in potential energy, which manifests as angle strain.
Torsional strain, on the other hand, occurs when there is a repulsion between electrons in bonds that don’t share an atom. This repulsion arises from the overlap of electron clouds, which increases the potential energy of the molecule. Think of it like trying to push two magnets together with the same poles facing each other. The repulsion you feel is similar to torsional strain.
A great example to visualize torsional strain is the staggered and eclipsed conformations of ethane. In the eclipsed conformation, the hydrogen atoms on adjacent carbon atoms are positioned directly in front of each other, leading to increased electron-electron repulsion and higher torsional strain. In contrast, the staggered conformation with a 60-degree dihedral angle between the hydrogen atoms reduces this repulsion and minimizes torsional strain.
To sum it up, angle strain arises from deviations in bond angles from their ideal values, while torsional strain arises from the repulsion between electron clouds in bonds that don’t share an atom. Both types of strain contribute to the overall energy of a molecule, affecting its stability and reactivity.
Does cyclohexane have torsional strain?
Angle strain arises when bond angles deviate from the ideal tetrahedral angle of 109.5 degrees. Think of it like trying to force a piece of paper into a smaller envelope – it causes stress and instability. Cyclohexane, with its six-membered ring, is able to achieve near-perfect tetrahedral angles, minimizing angle strain.
But what about torsional strain? This is a bit trickier. Torsional strain arises from the repulsion between electron clouds of bonds that are eclipsed – essentially, when they’re directly across from each other. In cyclohexane, there’s a unique conformation called the chair conformation that cleverly minimizes torsional strain.
Imagine a chair with its four legs and a backrest – that’s the shape of the cyclohexane molecule in its most stable conformation. In this chair conformation, the hydrogen atoms attached to the carbon atoms are staggered, not eclipsed, effectively minimizing torsional strain.
This is why cyclohexane is so stable. It avoids both angle and torsional strain, making it essentially as stable as a chain alkane.
See more here: What Is Torsional Strain? | Torsional Strain Vs Steric Strain
What is steric strain?
Think of it this way: imagine you’re building a model with small blocks. If you try to fit too many blocks together, they’ll start to bump into each other and push against each other. This is like steric strain!
Now, steric strain isn’t just about pushing and shoving; it can have a big impact on how molecules behave. It influences things like the shape of molecules, how quickly chemical reactions happen, and even how stable a molecule is.
For example, consider a molecule with large, bulky groups attached to it. These bulky groups might get in each other’s way, leading to steric strain. This can force the molecule into an unusual shape to try to minimize the repulsion. This change in shape can then affect the way the molecule interacts with other molecules.
Steric strain can also affect the speed of chemical reactions. Imagine you’re trying to fit a key into a lock. If the key is too big, it won’t fit, right? Similarly, if a molecule has large groups that create steric strain, it might be harder for another molecule to approach it and react.
So, steric strain might sound like a simple concept, but it has a big influence on the world around us. It’s a key factor in how molecules behave and interact, which plays a vital role in everything from the way drugs work to how plastics are made.
What is torsional strain in chemistry?
Imagine you’re twisting a rope. As you turn it, the strands of the rope bump into each other, right? Well, torsional strain is similar, but instead of a rope, we’re talking about the atoms and groups of atoms that make up a molecule.
When a molecule rotates around a sigma bond, the electrons in the bonds can bump into each other. This bumping, or repulsion, is what we call torsional strain. It’s like the strands of the rope getting in each other’s way.
Now, torsional strain is super important because it influences how a molecule wants to sit. Organic compounds, which are molecules made up of carbon, hydrogen, and sometimes other elements, try to avoid torsional strain. Think of it like a chair: you want to sit in a way that’s comfortable and avoids bumping your elbows on the person next to you. Molecules are the same way – they want to find a shape that minimizes this strain.
Let’s break it down further. Imagine you’re looking at a molecule with two groups of atoms attached to a central carbon atom. These groups can be anything like methyl groups, chlorine atoms, or even larger structures. As the molecule rotates, those groups pass by each other.
The amount of torsional strain depends on the angle between these groups. When they’re lined up, or eclipsed, the torsional strain is at its highest because the electrons in their bonds are bumping into each other. Think of it like two cars driving side-by-side at the same speed – they’re bound to hit each other. On the other hand, when the groups are staggered, or at an angle, the torsional strain is lower because the electrons have more space to move around. It’s like those two cars driving at a slight angle, allowing them to pass by each other without collision.
You can think of torsional strain as a kind of energy that molecules want to avoid. Just like a rock rolling downhill, a molecule will try to find the conformation, or shape, that has the lowest energy. This often means it will choose a conformation that minimizes torsional strain.
So, the next time you see a complex organic molecule, remember that torsional strain is playing a big role in determining its shape. It’s like a little game of molecular Tetris, with the molecules trying to fit their atoms together in a way that avoids bumping into each other.
What is the difference between steric hindrance and torsional strain?
Steric hindrance is a broader concept that describes the repulsion between electron clouds of atoms or groups that are close together. This repulsion leads to an increase in energy, making it harder for molecules to adopt certain conformations. It’s like trying to squeeze two large objects into a small space—they bump into each other, making it difficult to fit them comfortably.
Torsional strain, on the other hand, is a specific type of steric hindrance that occurs when groups on a molecule are eclipsed. Eclipsing happens when groups are directly aligned with each other, leading to maximum overlap of their electron clouds.
Imagine two groups on a molecule, like the blades of a pair of scissors. When the blades are open, they’re far apart, and there’s no significant strain. But when the blades are closed, they bump into each other, creating a significant strain.
Torsional strain is a more specific case of steric hindrance that specifically deals with eclipsed conformations.
Think of it this way:
Steric hindrance is like a general rule about avoiding crowded spaces.
Torsional strain is a specific example of crowding when things are directly on top of each other.
To illustrate the difference, let’s consider a simple example: Ethane (C2H6).
Ethane has two carbon atoms connected by a single bond. Each carbon atom is bonded to three hydrogen atoms. The molecule can rotate around the C-C bond, leading to different conformations.
One conformation is called the staggered conformation where the hydrogen atoms on each carbon atom are as far apart as possible. This conformation is the most stable because it minimizes steric hindrance.
Another conformation is called the eclipsed conformation where the hydrogen atoms on each carbon atom are directly aligned with each other. This conformation is less stable because it experiences torsional strain.
In summary, torsional strain is a specific type of steric hindrance that occurs when groups are eclipsed. While steric hindrance is a general concept that describes any repulsive interaction between atoms or groups, torsional strain specifically focuses on the repulsion between eclipsed groups.
What are the three types of steric and angular strain?
Angular strain pops up when the bond angles in a real molecule don’t match up with the ideal angles predicted by theories like VSEPR (Valence Shell Electron Pair Repulsion). Imagine a molecule like cyclopropane. It has a triangular shape with three carbon atoms, but the ideal bond angle for carbon is 109.5 degrees. In cyclopropane, the bond angles are forced to be 60 degrees, creating a lot of angular strain that makes the molecule less stable.
Torsional strain happens when atoms or groups of atoms attached to a molecule are rotated around a single bond. Think of a molecule like ethane, where two methyl groups (CH3) are attached to each other. When the methyl groups are eclipsed, they’re right next to each other, causing repulsion and making the molecule less stable. This is called torsional strain.
Steric strain, on the other hand, is all about how much space different parts of a molecule take up. It happens when bulky groups on a molecule get too close to each other, leading to repulsion and a less stable molecule. Consider a molecule like tert-butyl chloride (CH3)3CCl. The three methyl groups are large and take up a lot of space. When they are close to the chlorine atom, they experience steric strain and make the molecule less stable.
Here’s a deeper dive into each type of strain:
Angular Strain: Think of it like trying to squeeze a beach ball into a small box. The beach ball (our molecule) wants to spread out, but the box (our constraints) forces it into a smaller shape. This creates a lot of pressure (strain) in the beach ball. The same principle applies to molecules. When the bond angles are compressed or expanded from their ideal values, it causes a kind of “pressure” within the molecule, which is called angular strain. It’s like the molecule is trying to spring back to its ideal shape.
Torsional Strain: Imagine spinning a propeller. When the propeller blades are aligned, they create more drag and resistance, making it harder to spin. Similarly, when atoms or groups attached to a molecule are aligned in a way that causes repulsion, it creates torsional strain.
Steric Strain: This is like trying to fit multiple large objects into a small space. The objects (our bulky groups) will bump and jostle each other, trying to find some room to spread out. This bumping and jostling is steric strain. It’s a form of repulsion that happens when bulky groups on a molecule are too close together.
Understanding these types of strain is essential for predicting the stability of molecules, understanding their reactivity, and even designing new molecules with specific properties.
See more new information: countrymusicstop.com
Torsional Strain Vs Steric Strain: Understanding Conformational Energy
Have you ever wondered what makes molecules twist and turn, or how they manage to squeeze together in tight spaces? Well, you’re not alone! This is where the fascinating world of torsional strain and steric strain comes into play.
Think of molecules like little groups of friends hanging out, and the bonds between them are like the invisible threads holding them together. But, just like friends, molecules have their preferences too. Some don’t like to be too close, while others prefer a certain arrangement.
Let’s break down the difference between torsional strain and steric strain, diving into the nitty-gritty of how these forces affect the shapes and stability of molecules.
Torsional Strain: A Matter of Rotation
Imagine you’re holding a pencil and twisting it. You feel resistance, right? That’s kind of like what happens in molecules when their bonds rotate. Torsional strain, also known as dihedral strain, is the energy penalty a molecule pays when its bonds are twisted out of their most stable conformation.
The main culprit for this strain is the eclipsing of atoms or groups attached to adjacent carbons. This happens when they get too close, causing electron repulsion and making the molecule less stable.
For instance, let’s look at ethane, a simple molecule with two carbons and six hydrogens. When the hydrogens are in an eclipsed conformation, they are directly across from each other, leading to torsional strain.
Now, imagine rotating those carbons. You’ll find that the hydrogens are at a higher energy state when they are eclipsed compared to when they are staggered. This is because, in the staggered conformation, the hydrogens are further apart, minimizing electron repulsion and lowering the energy.
Think of it like this: the staggered conformation is like your friends standing comfortably, with some space between them. But the eclipsed conformation is like them all trying to squeeze into a small booth, leading to a bit of awkwardness (and maybe even a fight!).
Steric Strain: When Molecules Get Too Close
Remember how I said molecules have their preferences? Well, some molecules are just naturally bigger than others, and they can create problems when they get too close. This is where steric strain comes into play.
Steric strain is the energy penalty a molecule pays when atoms or groups get too close to each other. It’s like trying to squeeze a big beach ball into a small backpack. You’re going to have a tough time, and things are likely to get distorted.
In the world of molecules, this means that bulky groups attached to a molecule can make it harder for the molecule to adopt its most stable conformation. This can lead to a higher energy state and make the molecule less reactive.
Let’s consider cyclohexane, a ring-shaped molecule with six carbons. When cyclohexane is in its chair conformation, it’s pretty comfy. The bulky groups are all in equatorial positions, which gives them plenty of space.
But what happens when we try to stuff a bulky group into an axial position? It gets crowded and uncomfortable. This is because it’s trying to push through the other groups attached to the ring, leading to steric strain.
Think of it like trying to squeeze through a crowded doorway. If you’re carrying a big suitcase, you’re going to have a harder time than someone with a small handbag. The suitcase is like a bulky group in a cyclohexane ring, and the cramped doorway is like the axial position.
Torsional vs. Steric: A Quick Comparison
Here’s a quick breakdown of the key differences between torsional strain and steric strain:
| Strain | Cause | Effect |
|—|—|—|
| Torsional Strain | Rotation of bonds | Repulsion of electron clouds between atoms or groups on adjacent carbons |
| Steric Strain | Proximity of atoms or groups | Repulsion of electron clouds between atoms or groups on the same carbon |
Real-Life Examples: Where These Strains Matter
These strains are not just theoretical concepts. They play a crucial role in determining the shapes, stability, and reactivity of molecules, which has real-world implications.
For instance, torsional strain is a major factor in determining the conformations of proteins. Steric strain influences the way drugs bind to their targets, and it’s also important for understanding the stability of polymers.
Torsional strain and steric strain are key concepts in organic chemistry and biochemistry. They help us understand how molecules interact with each other and how these interactions influence their properties and behavior. So, the next time you see a molecule, remember the invisible forces that are keeping it in shape and determining its fate.
FAQs: Torsional Strain vs. Steric Strain
Here are some common questions about torsional strain and steric strain:
1. What is the difference between torsional and steric strain?
Torsional strain arises from the rotation of bonds, causing atoms or groups on adjacent carbons to get too close. This leads to electron repulsion. Steric strain, on the other hand, is caused by the proximity of atoms or groups on the same carbon, leading to electron repulsion between them.
2. How do these strains affect the stability of molecules?
Both torsional strain and steric strain increase the energy of a molecule, making it less stable. They can also make it more difficult for a molecule to undergo certain chemical reactions.
3. What are some examples of molecules that experience these strains?
Ethane and butane are classic examples of molecules that experience torsional strain. Cyclohexane and tert-butyl groups are good examples of molecules that experience steric strain.
4. How can we minimize these strains?
Torsional strain can be minimized by adopting a staggered conformation, where atoms or groups are as far apart as possible. Steric strain can be minimized by avoiding crowded conformations and choosing structures where bulky groups are in less hindered positions.
5. What are some applications of these concepts?
Torsional strain and steric strain are essential for understanding the structure and reactivity of molecules. They have implications in fields like drug design, material science, and catalysis.
6. Can these strains be measured?
Yes, torsional strain and steric strain can be measured using various experimental techniques like spectroscopy and calorimetry.
7. Why are these strains important in organic chemistry?
Torsional strain and steric strain are crucial in organic chemistry because they help us understand how molecules behave. They influence the conformations of molecules, their reactivity, and their physical properties.
8. Are there any exceptions to the rules of torsional and steric strain?
While the general principles of torsional strain and steric strain are well established, there are exceptions. Some molecules might exhibit unusual behavior due to factors like hydrogen bonding or unusual electron distribution.
9. How do these strains relate to other concepts in chemistry?
Torsional strain and steric strain are related to other concepts like bond angle strain, ring strain, and conformational analysis. They are all part of a broader understanding of molecular structure and reactivity.
10. What are some other examples of torsional and steric strain in everyday life?
Think about how a traffic jam creates a lot of stress and inefficiency. This is similar to steric strain in molecules. Likewise, trying to rotate a heavy door handle that is stuck is similar to torsional strain.
Understanding the concepts of torsional strain and steric strain will help you decipher the intricate dance of molecules, paving the way for a deeper appreciation of the fascinating world of chemistry.
What is the difference between steric strain and
Steric strain exists only in molecules who have four or more bonds, since steric strain is defined as the repulsion felt between atoms at four or more bonds separated from each other forced closer than their Chemistry Stack Exchange
What is the difference between steric and torsional strain?
We talk about torsional strain mainly when we draw Newman projection in the case of Eclipsed and Staggered. Steric strain is higher in the case of eclipsed Socratic
3.4. Types of Strain in Molecules – Introduction to
Learn the difference between torsional strain and steric strain, two types of strain that molecules may experience due to electronic repulsion. Torsional strain occurs when electron clouds from two atoms separated by three OPENPRESS.USASK.CA
Torsional and Steric Strain – Chemistry Steps
Learn how to identify and calculate the energy of torsional and steric strain in Newman projections of alkanes and cycloalkanes. See examples, definitions, and practice problems with solutions. Chemistry Steps
Understanding Torsional and Steric Strain in Chemistry
Learn the definitions, causes, and effects of torsional and steric strain, two types of energy associated with molecular rotation and spatial arrangement. Explore how mathematics warreninstitute.org
Steric Strain vs. Torsional Strain — What’s the Difference?
Steric Strain arises from the repulsion of atoms or groups due to their sizes; Torsional Strain results from the repulsion between electron pairs in eclipsed Ask Difference
8.2: Conformational Analysis – Chemistry LibreTexts
b) Torsional strain – Tendency of s-bonds to rotate in order to acquire a more stable conformation. c) Angle strain – Increase in potential energy due to bond angles being forced to depart from ideal values in cycloalkanes and other rings. libretexts.org
3.4: Stability of Cycloalkanes – Ring Strain – Chemistry LibreTexts
describe how the measurement of heats of combustion provides information about the amount of strain present in a cycloalkane ring. determine the relative stability Chemistry LibreTexts
Torsional Strain And Steric Strain
Strain In Organic Molecules | Angle Or Baeyer Strain | Steric Strain | Torsional Or Pitzer Strain
What Is Torsional Strain?
Explain Different Types Of Strain In Molecules | Stereochemistry | Organic Chemistry
Staggered, Eclipsed, Anti, And Gauche Conformers
Link to this article: torsional strain vs steric strain.
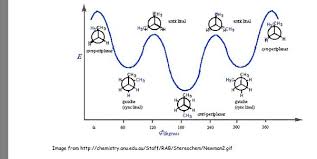
See more articles in the same category here: blog https://countrymusicstop.com/wiki