How does H2SO4 react with alkenes?
When alkenes react with concentrated sulfuric acid in the cold, alkyl hydrogensulfates are formed. For instance, ethene reacts to produce ethyl hydrogensulfate. The structure of the product molecule can be written as CH3CH2HSO4, but a more informative version shows how all the atoms are connected.
This reaction is an example of electrophilic addition. The sulfuric acid acts as an electrophile, meaning it is attracted to the electron-rich double bond of the alkene. The hydrogen ion from the sulfuric acid attacks the double bond, forming a carbocation. The carbocation then reacts with a sulfate ion to form the alkyl hydrogensulfate.
The reaction is an important step in the industrial production of ethanol. Ethene, a byproduct of cracking hydrocarbons, reacts with concentrated sulfuric acid to form ethyl hydrogensulfate. The ethyl hydrogensulfate is then hydrolyzed with water to produce ethanol.
This reaction is also used to synthesize other important organic compounds, such as diethyl ether and ethyl acetate.
I hope this explanation is clear and helpful. Feel free to ask if you have any more questions.
How do alkenes react with dilute acid?
You might be surprised to learn that alkenes, those hydrocarbons with a double bond, are quite reactive. In the presence of a dilute, aqueous acid, water can be added across the double bond, creating an alcohol. This reaction is called acid-catalyzed hydration. It’s a classic example of electrophilic addition, a common reaction mechanism in organic chemistry.
Here’s what happens:
1. Protonation: The acid, like sulfuric acid (H₂SO₄), donates a proton (H⁺) to the alkene. This creates a carbocation, a positively charged carbon atom.
2. Nucleophilic Attack: The carbocation is highly reactive, and water, which acts as a nucleophile, attacks it. The oxygen atom in water forms a bond with the carbocation, creating a protonated alcohol.
3. Deprotonation: Finally, a water molecule removes a proton from the protonated alcohol, yielding the alcohol product.
Let’s break this down with an example:
Imagine we have ethene (CH₂=CH₂), the simplest alkene, and dilute sulfuric acid. Here’s how the reaction would proceed:
1. The proton from the acid adds to one of the carbon atoms in the double bond, creating a carbocation.
2. Water, being a nucleophile, attacks the carbocation, forming a protonated alcohol.
3. Finally, a water molecule removes a proton from the protonated alcohol, forming ethanol (CH₃CH₂OH), our alcohol product.
The stability of the carbocation formed is crucial in determining the final product. If there are multiple possible carbocations, the more stable one will form. This is because a more stable carbocation is less likely to rearrange, leading to a more specific product.
The acid-catalyzed hydration of alkenes is a versatile reaction with various applications in the chemical industry. It’s used in the production of alcohols, which are essential building blocks for many other compounds.
So, the next time you see an alkene, remember its potential to react with dilute acid to form an alcohol – a testament to the fascinating world of organic chemistry!
What happens when ethene is treated with dil H2SO4?
Step 1: Formation of Ethyl Hydrogen Sulfate
The first step involves the addition of sulfuric acid to ethene. This results in the formation of ethyl hydrogen sulfate (CH₃CH₂OSO₃H). The reaction is reversible and requires heat to proceed.
Step 2: Hydrolysis of Ethyl Hydrogen Sulfate
The ethyl hydrogen sulfate formed in the first step is then hydrolyzed by water. This hydrolysis reaction produces ethanol (CH₃CH₂OH) and sulfuric acid.
Here’s a breakdown of the reaction:
Step 1:
C₂H₄ + H₂SO₄ ⇌ CH₃CH₂OSO₃H (reversible reaction, requires heat)
Step 2:
CH₃CH₂OSO₃H + H₂O → CH₃CH₂OH + H₂SO₄
Why is this reaction important?
This reaction is an essential step in the industrial production of ethanol. It demonstrates the electrophilic addition mechanism, a fundamental concept in organic chemistry.
Let’s take a closer look at the mechanism:
Electrophilic Attack: The double bond in ethene is rich in electrons, making it susceptible to electrophilic attack. The hydrogen ion (H⁺) from the sulfuric acid acts as the electrophile and attacks the double bond.
Carbocation Formation: The addition of the hydrogen ion forms a carbocation (CH₃CH₂⁺), an unstable intermediate.
Nucleophilic Attack: The sulfate ion (SO₄²⁻) from the sulfuric acid acts as a nucleophile and attacks the carbocation.
Ethyl Hydrogen Sulfate Formation: The nucleophilic attack results in the formation of ethyl hydrogen sulfate.
In summary:
Treating ethene with dilute sulfuric acid does not directly yield ethanol. Instead, it leads to the formation of ethyl hydrogen sulfate, which is subsequently hydrolyzed to produce ethanol. This process highlights the importance of electrophilic addition in organic chemistry and plays a key role in industrial ethanol production.
What does dilute H2SO4 do to an alcohol?
However, sulfuric acid is also a strong oxidizing agent, so it can also oxidize the alcohol. This means it can take electrons from the alcohol, leading to the formation of carbon dioxide (CO₂) and sulfur dioxide (SO₂). These gases are byproducts of the reaction and need to be removed to isolate the desired alkene.
The reaction of sulfuric acid with an alcohol can also produce a mass of carbon as a byproduct. This happens when the sulfuric acid acts as a dehydrating agent, removing water molecules from the alcohol, and leaving behind carbon.
Delving Deeper into the Oxidation of Alcohols with Dilute H₂SO₄
The oxidation of alcohols with dilute sulfuric acid is a complex process. It’s important to understand that the oxidation of alcohols depends on the type of alcohol and the concentration of the sulfuric acid. For instance, primary alcohols (where the hydroxyl group is attached to a primary carbon atom) can be oxidized to aldehydes, and then further to carboxylic acids, depending on the reaction conditions. Secondary alcohols (where the hydroxyl group is attached to a secondary carbon atom) can be oxidized to ketones. Tertiary alcohols (where the hydroxyl group is attached to a tertiary carbon atom) generally do not undergo oxidation under these conditions.
The oxidation of alcohols with dilute sulfuric acid is an example of a redox reaction – a reaction where electrons are transferred between reactants. In this case, the alcohol is being oxidized, meaning it is losing electrons. At the same time, the sulfuric acid is being reduced, meaning it is gaining electrons. This transfer of electrons is what drives the formation of carbon dioxide and sulfur dioxide.
While sulfuric acid is a powerful oxidizing agent, it’s important to note that the reaction conditions can influence the outcome. Factors like temperature, concentration, and the presence of other reactants can affect the rate and extent of the reaction.
Understanding the role of sulfuric acid in the oxidation of alcohols helps us to better understand the chemistry of these reactions and to predict the products that will be formed.
What happens when alkene reacts with dilute sulphuric acid?
Let’s break down the reaction a bit more. The sulfuric acid acts as a catalyst, meaning it speeds up the reaction without being consumed itself. It also provides the hydrogen ions (H+) needed for the reaction to occur. The alkene, with its double bond, is the reactant that undergoes the hydration.
The mechanism of this reaction involves the following steps:
1. Protonation: The alkene reacts with a hydrogen ion (H+) from the sulfuric acid, forming a carbocation. This is an unstable species with a positive charge on one of the carbon atoms.
2. Nucleophilic attack: A water molecule then acts as a nucleophile, attacking the carbocation and forming a new bond.
3. Deprotonation: The final step involves the removal of a hydrogen ion (H+) from the water molecule, resulting in the formation of the alcohol.
The type of alcohol produced depends on the structure of the alkene.
Primary alcohols are formed when the water molecule adds to a terminal carbon atom (a carbon atom at the end of the chain).
Secondary alcohols are formed when the water molecule adds to a carbon atom that is attached to two other carbon atoms.
Tertiary alcohols are formed when the water molecule adds to a carbon atom that is attached to three other carbon atoms.
The hydration of alkenes using dilute sulfuric acid is a common and important reaction in organic chemistry. It’s a versatile process that can be used to synthesize a wide variety of alcohols, which are important building blocks for many other organic compounds.
How do alkanes react with sulfuric acid?
Let’s delve deeper into why alkanes are so resistant to reaction with sulfuric acid. The reason lies in the nature of their chemical bonds. Alkanes consist solely of carbon and hydrogen atoms linked by strong single bonds. These bonds are very stable and require a considerable amount of energy to break. Sulfuric acid, while a strong acid, doesn’t possess enough energy to disrupt these robust bonds.
Furthermore, alkanes are non-polar molecules, meaning they have an even distribution of electrons. Sulfuric acid, on the other hand, is a polar molecule, with a concentration of positive charge on one end and negative charge on the other. This difference in polarity hinders any interaction between the two substances. The lack of polarity prevents sulfuric acid from effectively attacking the alkane’s bonds, further contributing to the absence of a reaction.
In essence, the combination of strong, stable bonds in alkanes and the inability of sulfuric acid to overcome these bonds due to polarity differences results in the observed lack of reactivity. This inertness of alkanes towards sulfuric acid is a fundamental concept in organic chemistry, highlighting the importance of understanding molecular structure and bonding in predicting chemical behavior.
What does H2SO4 do in a reaction?
H2SO4 acts as a dehydrating agent in the conversion of ethanol to ethane. It helps in removing a water molecule, specifically the -OH group from one carbon and -H from the other carbon.
Here’s how it works:
Ethanol (C2H5OH) is a primary alcohol. This means it has a hydroxyl group (-OH) attached to a primary carbon atom.
* When concentrated sulfuric acid is added to ethanol, the acid acts as a catalyst. It helps to remove a water molecule from the ethanol molecule, forming ethane (C2H6).
The Reaction:
C2H5OH → H2SO4 (conc.) → C2H6 + H2O
Understanding the Dehydration Process:
The dehydration reaction is a chemical reaction where a water molecule is removed from a molecule. This is a common reaction in organic chemistry, and sulfuric acid is often used as a catalyst for this reaction.
How Sulfuric Acid Works:
Sulfuric acid is a strong acid that is highly reactive. It is capable of protonating the hydroxyl group of ethanol, making it a better leaving group.
* The protonated hydroxyl group is then eliminated as water, leaving behind a carbocation.
* The carbocation is unstable and rapidly loses a proton to form ethane.
The Importance of Concentration:
Concentrated sulfuric acid is crucial for this reaction. The high concentration ensures that the acid can effectively protonate the hydroxyl group of ethanol.
In Summary:
H2SO4 is a powerful dehydrating agent that plays a critical role in the conversion of ethanol to ethane. Its ability to remove water molecules from ethanol drives the reaction forward and forms the desired product. The process involves protonation of the hydroxyl group, elimination of water, and formation of a carbocation, which ultimately leads to the formation of ethane.
See more here: How Do Alkenes React With Dilute Acid? | Reaction Of Alkene With Dilute H2So4
What happens when alkene reacts with dilute sulfuric acid?
The exciting part is that you can get different types of alcohols depending on where the water molecule attaches. It can form a primary, secondary, or tertiary alcohol.
Markovnikov’s rule helps us predict where that water molecule will attach. Basically, the hydrogen from the water molecule will attach to the carbon with the most hydrogen atoms already attached.
For example, if you have propene (CH3-CH=CH2), the hydrogen will attach to the CH2 end, giving you propan-2-ol (CH3-CH(OH)-CH3).
But, dilute sulfuric acid isn’t just hanging around; it acts as a catalyst in this reaction. It speeds things up without actually being consumed in the process. Pretty neat, right?
Let’s break down the reaction mechanism a bit more. The sulfuric acid protonates the alkene, forming a carbocation. The carbocation is then attacked by a water molecule, forming a protonated alcohol. Finally, the protonated alcohol loses a proton to give the final alcohol product.
So, there you have it! When an alkene reacts with dilute sulfuric acid, a hydration reaction occurs, leading to the formation of an alcohol. Markovnikov’s rule helps us predict the specific alcohol that will be formed. The sulfuric acid acts as a catalyst, speeding up the reaction.
Why is H2SO4 added in alkyne hydrolysis reaction?
Think of it like this: the alkyne is like a stubborn door that needs a little extra push to open. The mercury(II) sulfate acts as a key that helps unlock the door, and the sulfuric acid provides the energy needed to push it open. Together, they make the hydrolysis happen.
Let’s dive a little deeper into why H₂SO₄ is so important. The sulfuric acid acts as a proton donor, meaning it provides hydrogen ions (H⁺) to the reaction. These hydrogen ions are crucial because they help to stabilize the intermediate carbocation that forms during the hydrolysis process.
Here’s what happens:
1. The alkyne first reacts with the mercury(II) sulfate to form a mercury(II) alkoxy intermediate.
2. Then, the sulfuric acid comes into play. Its hydrogen ions attack the mercury(II) alkoxy intermediate, causing it to break down and form a carbocation.
3. Finally, water (H₂O) attacks the carbocation, resulting in the formation of a ketone.
Without the sulfuric acid, the carbocation would be too unstable and the reaction wouldn’t proceed. So, you can see why H₂SO₄ is essential for this reaction to happen.
In essence, the H₂SO₄ acts as a catalyst to facilitate the hydrolysis of the alkyne by providing the necessary hydrogen ions. This crucial role is what makes it possible to convert the alkyne to a ketone in a controlled manner.
How does sulfuric acid react with unsymmetrical alkenes?
Now, to understand the different ways we can write the formula for the product, it’s crucial to visualize the structure of sulfuric acid. We’ll use this visual to help you see how the atoms rearrange during the reaction. It’s important to remember this reaction is typical for unsymmetrical alkenes, meaning the two carbon atoms on either side of the double bond have different groups attached to them.
Here’s how it works:
1. Protonation: The sulfuric acid acts as a proton donor (acid) and donates a proton (H+) to the alkene. This proton attaches to one of the carbon atoms in the double bond, forming a carbocation.
2. Carbocation Formation: The carbon atom that receives the proton becomes positively charged, forming a carbocation. This carbocation is unstable and highly reactive.
3. Nucleophilic Attack: The sulfuric acid molecule (HSO4-) acts as a nucleophile, meaning it is attracted to the positive charge of the carbocation. The sulfuric acid molecule attacks the carbocation, forming a new bond with the carbon atom.
4. Product Formation: The final product is an alkyl hydrogen sulfate, which is a compound that contains an alkyl group (R-) attached to a hydrogen sulfate group (HSO4-).
Example:
Let’s consider the reaction of sulfuric acid with propene (CH3CH=CH2), which is an unsymmetrical alkene.
1. Protonation: The proton from the sulfuric acid attaches to the middle carbon atom in the propene molecule. This forms a carbocation, with a positive charge on the middle carbon.
2. Nucleophilic Attack: The sulfuric acid molecule attacks the carbocation, forming a new bond with the positively charged carbon atom.
3. Product Formation: The product of this reaction is isopropyl hydrogen sulfate (CH3CH(OSO3H)CH3).
Understanding the Structure of Sulfuric Acid
The way we write the formula for the product is based on the structure of sulfuric acid. Here’s a quick overview of sulfuric acid’s structure:
Sulfuric acid (H2SO4): The central sulfur atom is surrounded by four oxygen atoms. Two of these oxygen atoms are bonded to hydrogen atoms, forming hydroxyl groups (OH). The other two oxygen atoms are bonded to the sulfur atom with a double bond.
By understanding the structure of sulfuric acid, we can see how the atoms rearrange during the reaction. This helps us to write the formula for the product correctly.
In Conclusion
This reaction is essential in many organic chemistry reactions and is a vital process for understanding the chemistry of alkenes and sulfuric acid.
See more new information: countrymusicstop.com
Reaction Of Alkene With Dilute H2So4 | How Does H2So4 React With Alkenes?
Hey there, chemistry enthusiasts! Today, we’re diving into the fascinating world of alkene reactions, specifically with dilute sulfuric acid (H2SO4). This reaction is a classic example of electrophilic addition, a fundamental concept in organic chemistry. Let’s break down what happens and why it’s so important.
Understanding the Players
First things first, let’s get familiar with our key players:
Alkenes: These are hydrocarbons with a carbon-carbon double bond. Think of them as molecules with a “hungry” double bond that’s ready to react.
Dilute H2SO4: This is sulfuric acid diluted with water. In this reaction, the acid acts as a catalyst, which means it speeds up the reaction without being consumed itself.
The Electrophilic Attack
When you mix an alkene with dilute H2SO4, the magic begins. Here’s how it unfolds:
1. Protonation: The first step involves the protonation of the alkene. The hydrogen ion (H+) from the sulfuric acid attacks the pi bond of the alkene. This pi bond, which is a weaker bond compared to the sigma bond, is more susceptible to attack.
2. Carbocation Formation: The protonation of the alkene forms a carbocation – a positively charged carbon atom. This carbocation is very unstable and wants to get rid of its positive charge.
3. Nucleophilic Attack: Now, the water molecule acts as a nucleophile, meaning it has a lone pair of electrons it wants to donate. This water molecule attacks the carbocation, forming a new C-O bond.
The End Product: An Alcohol
The final product of this reaction is an alcohol. The position of the hydroxyl group (-OH) in the alcohol is determined by the stability of the carbocation formed in the intermediate step. The more stable the carbocation, the more likely it is to form.
Here’s an example:
Let’s say you react propene (CH3CH=CH2) with dilute H2SO4. The product will be propan-2-ol (CH3CH(OH)CH3).
Why does this happen?
The carbocation formed from propene is more stable if the positive charge is on the secondary carbon (the carbon attached to two other carbons). This is because the alkyl groups attached to the carbocation can donate electron density, stabilizing the positive charge.
Factors Affecting the Reaction
Alkene Structure: The structure of the alkene plays a crucial role. For example, terminal alkenes (alkenes with the double bond at the end of the chain) are more likely to react with dilute H2SO4 than internal alkenes (alkenes with the double bond within the chain).
Concentration of H2SO4: The concentration of H2SO4 affects the rate of the reaction. A more concentrated solution will lead to a faster reaction.
Temperature: Higher temperatures generally lead to faster reaction rates.
Applications of the Reaction
The reaction of alkenes with dilute H2SO4 has many practical applications, including:
Industrial production of alcohols: This reaction is used in the production of various alcohols, like ethanol and isopropanol.
Synthesis of pharmaceuticals: This reaction is crucial in the synthesis of many pharmaceuticals.
Organic Chemistry Research: This reaction is a valuable tool for organic chemists studying the behavior of alkenes.
FAQs
Q1: What is the difference between dilute and concentrated H2SO4 in this reaction?
A1: The concentration of H2SO4 influences the reaction. Dilute H2SO4 predominantly leads to alcohol formation, while concentrated H2SO4 can lead to other products like ethers and alkenes.
Q2: What are the side reactions that can occur in this reaction?
A2: Side reactions can occur, especially with concentrated H2SO4. These include dehydration, where water is removed from the alcohol to form an alkene, and rearrangement, where the carbocation rearranges to form a more stable carbocation.
Q3: Can other acids be used instead of H2SO4?
A3: Yes, other strong acids, like hydrochloric acid (HCl) and hydrobromic acid (HBr), can also be used for this reaction.
Q4: What are the safety precautions that need to be taken when working with dilute H2SO4?
A4: Always wear appropriate safety gear, including gloves, goggles, and a lab coat. Remember that sulfuric acid is corrosive and can cause severe burns.
Wrapping It Up
That’s a quick look at the reaction of alkenes with dilute H2SO4! As you can see, this reaction is an excellent example of how simple reactions can lead to complex and useful products. I hope this article has given you a better understanding of the chemistry behind this reaction.
If you’re interested in learning more about alkene reactions, or organic chemistry in general, don’t hesitate to check out other resources online or consult your chemistry textbook. Happy learning!
Reactions of Alkenes with Sulfuric Acid – Chemistry
Alkenes react with concentrated sulfuric acid in the cold to produce alkyl hydrogensulphates. For example, ethene reacts to give ethyl hydrogensulphate. \[ \ce{CH_2=CH_2 + H_2SO_4 \rightarrow Chemistry LibreTexts
alkenes and sulphuric (sulfuric) acid – chemguide
Alkenes react with concentrated sulphuric acid in the cold to produce alkyl hydrogensulphates. Ethene reacts to give ethyl hydrogensulphate. The structure of the chemguide
Addition of Sulfuric acid to Alkenes – Chemistry LibreTexts
The reaction with ethene. Alkenes react with concentrated sulfuric acid in the cold to produce alkyl hydrogensulfates. Ethene reacts to give ethyl hydrogensulfate. Chemistry LibreTexts
Alkene + H2SO4 + H2O – YouTube
This organic chemistry video tutorial explains how to find the major product for the reaction of an alkene with H2SO4 and H2O. It also explains how to write… YouTube
10.3: Reactions of Alkenes- Addition of Water (or Alcohol) to
The acid most commonly applied to catalyze this reaction is dilute aqueous solution of sulfuric acid (H 2 SO 4 ). Sulfuric acid dissociates completely in aqueous Chemistry LibreTexts
Reactions of Alkenes – Rutgers University
The reaction of dilute acid to hydrate alkenes is not a fantastic practical route due to insolubility problems, and typically two other indirect approaches are used. (1) Addition crab.rutgers.edu ARCHIVE
Ch15: Hydration of alkenes – Faculty of Science
Reaction type: Electrophilic Addition. Summary. When treated with aq. acid, most commonly H 2 SO 4, alkenes form alcohols. Regioselectivity predicted by Markovnikov’s ucalgary.ca
Hydration (video) | Alkene reactions | Khan Academy
So the way to control your equilibrium is if you want to go to the right, you just dilute your sulfuric acid. You add more water to it, which would increase this concentration. If you want to shift the equilibrium to the left, you decrease your concentration of water, Khan Academy
Reaction between Sulphuric Acid and Alkenes – ChemiPhys
The sulphuric acid reacts with the alkene to produce a alkyl hydrogensulphate. This is responsible for the increase in the viscosity of the mixture and the colour change. Two chemiphys.com
Alkene + H2So4 + H2O
Dil/Conc H²So4 With Alkene || Reaction Of Alcohol With Conc H2So4 || H2So4 Reagents And Application
Alkene + Kmno4 Reaction
A Level Chemistry Revision \”Reaction Between Alkenes And Sulfuric Acid\”
Potassium Metal Reacts With Concentrated Sulfuric Acid
Link to this article: reaction of alkene with dilute h2so4.
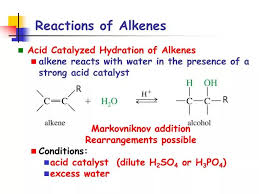
See more articles in the same category here: blog https://countrymusicstop.com/wiki