What is the reaction of alpha beta unsaturated ketone?
Alpha beta unsaturated ketones are a special type of ketone molecule that has a double bond between the alpha and beta carbons. This double bond gives them unique reactivity.
Here’s how the reaction works:
1. DHA (dehydroascorbic acid) reacts with an aromatic aldehyde. This initial reaction forms the alpha beta unsaturated ketone.
2. The newly formed alpha beta unsaturated ketone then reacts with o-ATP (ortho-aminophenylthiophosphoric acid). This reaction creates two distinct compounds: dihydro-1,5-benzothiazepines and dihydrobenzothiazines.
These reactions are important for understanding the chemistry of alpha beta unsaturated ketones and their potential applications in various fields.
Now, let’s delve deeper into the alpha beta unsaturated ketones and their reactivity.
The reactivity of alpha beta unsaturated ketones stems from the presence of the double bond and the carbonyl group. The double bond makes the molecule susceptible to electrophilic attack, while the carbonyl group offers opportunities for nucleophilic addition.
The electrophilic attack can occur at either the alpha or beta carbon. The addition of an electrophile to the beta carbon is particularly important, as it leads to the formation of a new carbon-carbon bond. This type of reaction is known as Michael addition.
Nucleophilic addition can occur at the carbonyl group of the alpha beta unsaturated ketone. This reaction can lead to the formation of a new carbon-oxygen bond.
The reactions of alpha beta unsaturated ketones are widely used in organic synthesis. They are employed in the preparation of various organic compounds, including natural products and pharmaceuticals.
For example, alpha beta unsaturated ketones are key intermediates in the synthesis of vitamin A. They also play a crucial role in the synthesis of anti-inflammatory drugs and anticancer agents.
Understanding the reactivity of alpha beta unsaturated ketones is essential for researchers in chemistry, pharmacy, and other related fields. Their unique properties make them versatile building blocks for various organic molecules with potential applications in medicine, agriculture, and beyond.
What is the hydrogenation of alpha beta unsaturated ketones?
Let’s break it down. Alpha, beta-unsaturated ketones have a special structure: they have a carbonyl group (C=O) next to a carbon-carbon double bond (C=C). This arrangement makes them reactive, and they can undergo hydrogenation, which is the addition of hydrogen (H2).
During hydrogenation, the catalyst, usually a metal like palladium or platinum, helps add hydrogen atoms to the molecule. If the hydrogenation occurs at the C=O bond, you get an unsaturated alcohol (UA). This is because the carbonyl group is reduced to a hydroxyl group (OH), while the carbon-carbon double bond remains intact.
On the other hand, if the hydrogenation happens at the C=C bond, the carbon-carbon double bond gets reduced to a single bond, resulting in a saturated ketone/aldehyde (SK/SAL). The carbonyl group remains untouched in this scenario.
Think of it like this: You have a double-decker bus with a ladder on top. The hydrogen atoms are like passengers trying to get onto the bus. If they climb the ladder and get onto the top deck (C=O bond), you’ll have a bus with passengers on the top, but still two levels (unsaturated alcohol). But if they climb onto the bottom deck (C=C bond), the bus will become a single-decker bus with passengers (saturated ketone/aldehyde).
The choice of catalyst and reaction conditions plays a crucial role in determining which product you’ll get. Sometimes, you might even get a mixture of both products! Understanding the factors that influence the direction of the reaction is important for controlling the outcome and getting the desired product.
What are the examples of enone?
Enones are special molecules with a double bond next to a carbonyl group. They are quite common and are often created through chemical reactions like the aldol condensation or the Knoevenagel condensation.
These reactions involve combining molecules in specific ways to build the enone structure. For instance, mesityl oxide, a dimer of acetone, is produced by condensing two acetone molecules. Similarly, the trimers, phorone and isophorone, are made by combining three acetone molecules.
It’s interesting to see how these basic building blocks can lead to a variety of different enones.
These enones are used in many applications. For example, mesityl oxide is a versatile building block for organic synthesis, and phorone is used in the production of polymers and resins. Isophorone is a solvent used in paints and coatings.
But that’s not all! Enones have a fascinating history too. They were first discovered in the early 19th century and quickly gained attention for their unique reactivity.
The study of enones led to significant advances in organic chemistry, including the development of new synthetic methods and the understanding of reaction mechanisms. The discovery of enones paved the way for understanding and developing many other important organic molecules, contributing greatly to the advancement of organic chemistry.
Why is it called alpha beta unsaturated?
You’re probably familiar with the idea of saturation in chemistry. It refers to how many hydrogen atoms are attached to a carbon atom. A saturated carbon has the maximum number of hydrogens it can hold. For example, in methane (CH4), the carbon atom is fully saturated with four hydrogen atoms.
Now, when we introduce a double bond between two carbon atoms, we effectively remove two hydrogen atoms. This is what we call unsaturation. We’ve replaced two single bonds with a single double bond. The same principle applies when we have a carbonyl group (C=O) attached to the carbon chain.
So, why alpha beta unsaturated? Well, it all comes down to labeling. The carbon atom directly attached to the carbonyl group is labeled alpha, and the next carbon atom is labeled beta. This labeling system helps us quickly identify the positions of functional groups in a molecule, especially in organic chemistry where molecules can get quite complex.
Therefore, when we have a carbonyl group directly attached to a double bond, we are essentially removing two hydrogen atoms from the alpha and beta carbon atoms, making the molecule unsaturated. This is why we call it an alpha beta unsaturated ketone!
Think of it like this: the double bond creates a “gap” where we could potentially add hydrogen atoms. This “gap” is associated with the alpha and beta positions, leading to the name alpha beta unsaturated.
Let’s consider a simple example:
Acetone (CH3COCH3) is a saturated ketone because it has no double bond next to the carbonyl group.
Methyl vinyl ketone (CH3COCH=CH2), on the other hand, is an alpha beta unsaturated ketone because it has a double bond next to the carbonyl group, removing hydrogen atoms from the alpha and beta positions.
You can also think of alpha beta unsaturated ketones as a type of conjugated system. This means that the carbonyl group and the double bond are connected by a single bond, allowing for electron delocalization across the entire system. This conjugation contributes to the unique reactivity and stability of alpha beta unsaturated ketones.
Understanding the concept of alpha beta unsaturation is crucial for comprehending the reactivity and properties of many organic compounds. It plays a key role in various chemical reactions, making it a fundamental concept in organic chemistry.
What are alpha beta unsaturated aldehydes used for?
Alpha beta unsaturated aldehydes are versatile molecules with a unique structure that makes them valuable in organic synthesis. They contain a carbon-carbon double bond (C=C) adjacent to a carbonyl group (C=O), giving them a reactive nature.
Here’s how they’re used to produce azolium dienolate intermediates:
NHC Catalysts:N-Heterocyclic carbenes (NHCs) are powerful catalysts that can activate alpha beta unsaturated aldehydes. Think of them as tiny helpers that make the reaction go faster and more efficiently.
Reaction Mechanism: The NHC catalyst reacts with the alpha beta unsaturated aldehyde, leading to the formation of an azolium dienolate intermediate. This intermediate is a crucial stepping stone in the overall reaction process.
Applications: This strategy is a powerful tool for γ-functionalization of unactivated C–H bonds. In simpler terms, it allows chemists to introduce new functional groups onto a molecule at a specific position that wouldn’t normally react easily. This opens up possibilities for creating complex molecules with specific properties.
Think of it like this: Imagine you have a simple building block (the alpha beta unsaturated aldehyde) and you want to add a new room (the functional group). The NHC catalyst acts as a skilled construction worker, helping you build the room in a specific spot (the γ-position) on your building block.
Here’s an analogy to help you visualize it:
Imagine you have a long chain of building blocks, each representing a carbon atom. You want to add a new piece to this chain, but it’s difficult because the chain is already connected. The alpha beta unsaturated aldehyde is like a special building block with a “handle” (the carbonyl group) and a “hook” (the double bond) that can be used to attach the new piece. The NHC catalyst acts as a tool that helps you connect the new piece to the chain using the handle and the hook. The result is a modified chain with a new building block attached to it.
In a nutshell, the use of alpha beta unsaturated aldehydes with NHC catalysts allows chemists to perform valuable transformations, creating new and useful compounds. This approach has opened new doors in organic synthesis, paving the way for the development of new drugs, materials, and other exciting products.
Does hydrogenation affect ketones?
The use of asymmetric hydrogenation to create secondary alcohols from ketones has become an increasingly popular method in organic chemistry. This is due to the fact that it is a highly efficient and selective reaction, leading to the production of high yields of optically active secondary alcohols. These alcohols are valuable intermediates in the synthesis of various biologically active compounds, including pharmaceuticals, perfumes, and agrochemicals.
Let’s break down how asymmetric hydrogenation works in the context of ketones:
Ketones are organic compounds with a carbonyl group (C=O) attached to two carbon atoms. The carbonyl group in a ketone is a reactive site, which means it can undergo various chemical reactions.
Hydrogenation of a ketone involves the addition of hydrogen atoms to the carbonyl group. This process results in the reduction of the carbonyl group to a hydroxyl group (OH).
Asymmetric hydrogenation uses a chiral catalyst to control the stereochemistry of the reaction, meaning that it determines the specific spatial arrangement of the atoms in the product. This process results in the formation of a single enantiomer of the secondary alcohol, meaning that it is a single mirror image of the molecule.
Scheme 1, a visual representation of the process, can be used to understand the overall reaction of asymmetric hydrogenation of ketones. It shows how the ketone is reacted with hydrogen in the presence of a chiral catalyst to form an enantiomerically pure secondary alcohol.
It is important to note that the choice of chiral catalyst is crucial for the success of asymmetric hydrogenation. The catalyst must be able to effectively distinguish between the two faces of the carbonyl group and preferentially add hydrogen to one side. This ensures that the desired enantiomer of the secondary alcohol is produced.
The asymmetric hydrogenation of ketones is a versatile and powerful tool in organic synthesis, enabling the production of enantiomerically pure secondary alcohols. These alcohols are essential building blocks for a wide range of important compounds, further highlighting the significance of this reaction in the field of chemistry.
What is the product of the hydrogenation of ketones?
Let’s break down the process a bit further. Ketones are organic compounds that contain a carbonyl group (C=O), which is a carbon atom double-bonded to an oxygen atom. When a ketone is hydrogenated, the hydrogen atoms add to the carbonyl group, breaking the double bond and forming a single bond between the carbon and oxygen. This process essentially converts the carbonyl group into a hydroxyl group (-OH), which is the characteristic functional group of alcohols.
For example, consider the hydrogenation of propanone (acetone), a common ketone. In the presence of a catalyst like nickel or palladium, propanone reacts with hydrogen gas to yield propan-2-ol (isopropyl alcohol).
This transformation from a ketone to an alcohol is a fundamental reaction in organic chemistry and has various applications in different industries. For example, the hydrogenation of ketones is used in the production of various alcohols, including those used in pharmaceuticals, cosmetics, and other industrial applications.
What is selective hydrogenation of unsaturated ketones?
Traditionally, achieving this selective hydrogenation has relied heavily on precious metals. These metals, often used as catalysts, are effective at promoting the desired reaction, but they can be costly and have environmental concerns. Transfer hydrogenation is a common method used in this context, where a hydrogen donor molecule transfers hydrogen atoms to the unsaturated ketone. This process typically involves the use of precious metal catalysts, such as ruthenium, rhodium, or palladium, which facilitate the transfer of hydrogen. While this approach has been successful, the search for more sustainable and cost-effective alternatives is ongoing.
Let’s dive a bit deeper into transfer hydrogenation and the role of precious metals. The process involves two key steps. First, the hydrogen donor molecule, often a secondary alcohol, gets oxidized, releasing a hydride ion (H-). This hydride ion is then transferred to the α,β-unsaturated ketone, resulting in the 1,4-addition of hydrogen to the double bond. Precious metals play a crucial role in this process by acting as catalysts. They facilitate the transfer of hydrogen by providing a surface for the reaction to occur and by lowering the activation energy required for the reaction to proceed.
The use of precious metals in transfer hydrogenation has been instrumental in developing efficient methods for selective hydrogenation, but there are drawbacks. These metals can be expensive, and their availability is limited, making them less desirable from a cost and sustainability perspective. Additionally, some of these metals can be toxic, raising concerns about their impact on the environment. These factors have spurred researchers to explore alternative catalysts and methodologies to achieve the desired selectivity without relying on precious metals. The development of more sustainable and cost-effective approaches remains a key area of research in this field.
See more here: What Is The Hydrogenation Of Alpha Beta Unsaturated Ketones? | Alpha Beta Unsaturated Ketone Reduction
See more new information: countrymusicstop.com
Alpha Beta Unsaturated Ketone Reduction: Strategies And Applications
Alright, let’s talk about alpha beta unsaturated ketone reduction, shall we? This is a reaction that’s pretty important in organic chemistry, and it’s also a great example of how we can use different reagents to get different results.
So, what exactly is an alpha beta unsaturated ketone? It’s a molecule with a ketone group (C=O) attached to a carbon-carbon double bond. We call it “alpha beta” because the ketone group is directly attached to the alpha carbon (the carbon next to the carbonyl group), and the double bond is attached to the beta carbon (the carbon two positions away from the carbonyl group).
Now, reducing these ketones is all about adding hydrogen atoms across the double bond. But here’s the catch: there are multiple ways to do this, each with its own set of advantages and disadvantages.
The Different Methods
The most common methods for reducing alpha beta unsaturated ketones are:
1. Catalytic Hydrogenation: This is the classic way to reduce double bonds. You use a metal catalyst, like platinum or palladium, and hydrogen gas (H2) to add hydrogen atoms across the double bond. This method is usually quite efficient, but it can also lead to over-reduction, where the ketone group is also reduced to an alcohol.
2. Hydride Reduction: Here, we use reducing agents like sodium borohydride (NaBH4) or lithium aluminum hydride (LiAlH4). These powerful reagents can attack the carbonyl group and the double bond. However, they are often too strong for selective reduction.
3. 1,4-Addition: Here’s where things get interesting. This method involves attacking the double bond, not the carbonyl group. We use reagents like Grignard or organolithium to add a new carbon-carbon bond. This strategy allows us to create a new chiral center in the molecule.
Factors Affecting the Outcome
The outcome of alpha beta unsaturated ketone reduction depends on a few key factors:
The Reagent: Different reducing agents have different reactivity and selectivity. Sodium borohydride, for example, tends to reduce only the carbonyl group, while lithium aluminum hydride can also reduce the double bond.
The Reaction Conditions: The solvent, temperature, and pressure can all influence the outcome of the reaction.
Applications of Alpha Beta Unsaturated Ketone Reduction
So why is all this important? Alpha beta unsaturated ketone reduction has a number of applications in organic chemistry, including:
Synthesis of Pharmaceuticals: Many pharmaceuticals are synthesized through this process.
Natural Product Synthesis: This is a vital tool for creating complex molecules found in nature.
Polymers: Reduction reactions can be used to create polymers with specific properties.
FAQs
Q: What are the different types of alpha beta unsaturated ketones?
A: There are several types of alpha beta unsaturated ketones, including:
Cyclic ketones: These have a ring structure containing the ketone and double bond.
Acyclic ketones: These have an open-chain structure.
Substituted ketones: These have various substituents attached to the ketone and double bond.
Q: Why is the double bond in an alpha beta unsaturated ketone special?
A: The double bond in an alpha beta unsaturated ketone is special because it’s conjugated with the carbonyl group. This means that the electrons in the double bond and the carbonyl group can interact, leading to enhanced reactivity.
Q: Can I use different reagents to reduce an alpha beta unsaturated ketone?
A: Yes, you can use different reagents to reduce an alpha beta unsaturated ketone, but the choice of reagent will determine the outcome. Some reagents will selectively reduce the carbonyl group, while others will reduce both the carbonyl group and the double bond.
Q: How can I tell if an alpha beta unsaturated ketone has been reduced?
A: You can tell if an alpha beta unsaturated ketone has been reduced by looking for the following changes:
The double bond disappears.
The carbonyl group may or may not be reduced depending on the reagent.
Q: What are the advantages and disadvantages of the different reduction methods?
A:
| Method | Advantages | Disadvantages |
|—|—|—|
| Catalytic Hydrogenation | * High yield * Relatively inexpensive | * Can over-reduce * Requires specialized equipment |
| Hydride Reduction | * Versatile * Can be used with a variety of functional groups | * Can over-reduce * Sensitive to moisture * Can be difficult to control |
| 1,4-Addition | * Selective * Allows for the creation of new chiral centers | * Can be challenging to control * Not always applicable |
Q: What are some other things I should know about alpha beta unsaturated ketone reduction?
A:
Stereochemistry: The reduction of an alpha beta unsaturated ketone can produce stereoisomers (different spatial arrangements of atoms).
Reaction Mechanisms: Understanding the mechanism of the reaction is essential for predicting the outcome and optimizing the reaction conditions.
Applications:Alpha beta unsaturated ketone reduction has many applications in organic synthesis, drug discovery, and material science.
Remember, the best method for reducing an alpha beta unsaturated ketone will depend on your specific needs and the desired outcome. By carefully considering the factors discussed above, you can choose the best method for your specific reaction.
Chemoselective Luche-Type Reduction of α,β-Unsaturated
The chemoselective reduction of α,β-unsaturated ketones by use of an economic and readily available Mg catalyst has been developed. Excellent yields for a ACS Publications
Highly Selective Reduction of α, β‐Unsaturated
An efficient and green protocol for highly selective reduction of α,β-unsaturated aldehydes and ketones at room temperature is Chemistry Europe
Chemoselective Conjugate Reduction of α,β-Unsaturated
Now we have found that a variety of α,β-unsaturated ketones, even without other electron-withdrawing functional groups, could be reduced on the alkenic double ACS Publications
Electrochemical 1,4-reduction of α,β-unsaturated ketones with
A sustainable, chemoselective 1,4-reduction of α,β-unsaturated ketones by means of an electrochemical method is presented, wherein the extremely inexpensive RSC Publishing
Chemoselective and metal-free reduction of α,β
General procedure for chemoselective reduction of (hetero)arylidene acetones 2a–o. A 25 mL round-bottom flask equipped with a stir bar and a condenser was charged with α,β-unsaturated ketone 2 (0.3 mmol), RSC Publishing
Chemoselective reduction of α,β-unsaturated ketones to allylic
Herein, we describe an ever more expedient, green and general method for the chemoselective reductions of exo- and endo-α,β-unsaturated cycloketones and acyclic RSC Publishing
1,2- Versus 1,4-Asymmetric Reduction of Ketones | SpringerLink
Reduction of α,β-unsaturated ketones generally can give rise to a variety of products, of which chiral allylic alcohols (1,2-reduction) and saturated ketones (1,4 Springer
Electrochemical 1,4-reduction of α,β-unsaturated ketones
A sustainable, chemoselective 1,4-reduction of α,β-unsaturated ketones by means of an electrochemical method is presented, wherein the extremely inexpensive PubMed
Synthesis of α,β-unsaturated ketones through nickel-catalysed
Transition metal-catalysed hydroacylation of alkynes using aldehydes is an atom-economical route to access α,β-unsaturated ketones, but typically requires Nature
14.01 Alpha,Beta-Unsaturated Carbonyl Compounds
Direct 1,2 Addition In Alpha Beta Unsaturated Ketones Aldehydes And Carboxylic Acid Derivatives
Reduction Of Unsaturated Ketones
Aldehydes And Ketones
Nucleophilic Addition Reaction Mechanism, Grignard Reagent, Nabh4, Lialh4, Imine, Enamine, Reduction
Link to this article: alpha beta unsaturated ketone reduction.
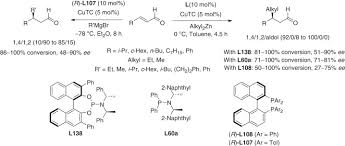
See more articles in the same category here: blog https://countrymusicstop.com/wiki