What is the relationship between nucleophilicity and leaving group ability?
Nucleophilicity measures how well a species, called a nucleophile, can donate electrons and form a new bond. The stronger the nucleophile, the more readily it will attack an electrophile (electron-loving species). Think of it like a “bond-making” ability.
On the other hand, leaving group ability refers to how easily a species, called a leaving group, departs from a molecule, taking its electrons with it. The better the leaving group, the more stable it is after breaking away, which makes it easier for the reaction to proceed. Think of it like a “bond-breaking” ability.
You might be thinking, “How are these two connected?” Well, the key lies in the strength of the bond between the carbon atom and the leaving group. Stronger bonds mean that the leaving group will not readily depart, making it a poor leaving group. This also means that the nucleophile will have a tougher time attacking the carbon atom, reducing its nucleophilicity.
Weak bonds, on the other hand, allow the leaving group to easily detach, making it a good leaving group. This makes the carbon atom more susceptible to nucleophilic attack, leading to a higher nucleophilicity.
Let’s dive a bit deeper. Imagine a tug-of-war between a nucleophile and a leaving group. The strength of the nucleophile determines how hard it can pull, and the strength of the leaving group determines how hard it can resist being pulled away.
A strong nucleophile will easily overcome the resistance of a weak leaving group, leading to a fast reaction. A weak nucleophile will struggle against a strong leaving group, resulting in a slow or non-existent reaction.
Here’s a simple analogy: Imagine a game of tug-of-war between two teams. The team with the stronger players (strong nucleophile) will easily pull the rope away from the weaker team (weak leaving group). Conversely, a weaker team (weak nucleophile) will struggle to pull against a stronger team (strong leaving group).
This interplay between nucleophilicity and leaving group ability is crucial for understanding a wide range of chemical reactions. Whether you’re studying organic chemistry, biochemistry, or even pharmaceuticals, grasping these concepts will be vital in your journey!
Is a stronger nucleophile a better leaving group?
A stronger nucleophile is not necessarily a better leaving group.
Think of it this way: reactions favor going from a stronger species to a weaker species. This principle applies to both acid-base reactions and substitution/elimination reactions.
In a substitution or elimination reaction, we want the leaving group to be a weaker base than the nucleophile. This makes the reaction more likely to proceed. Why? Because a weaker base is less likely to grab back the electron pair it lost, allowing the reaction to move forward.
Let’s break down why a strong nucleophile is not necessarily a good leaving group.
Nucleophiles: These are species that are attracted to positively charged centers and donate electron pairs. A stronger nucleophile is more likely to donate its electron pair, making it more reactive.
Leaving Groups: These are groups that depart from a molecule, taking their bonding electrons with them. A good leaving group is one that leaves readily, without wanting to grab those electrons back. This means it’s typically a weak base.
Why are strong nucleophiles not good leaving groups?
A strong nucleophile is a species that loves to donate electrons. Imagine a strong base – it’s like a clingy friend who doesn’t want to let go. A strong nucleophile would also be a strong base, meaning it would love to snatch the electron pair back from the leaving group.
For instance, hydroxide (OH-) is a strong base and a strong nucleophile, but it’s a very poor leaving group because it wants to hold onto its electrons. In contrast, the chloride ion (Cl-) is a weaker base and a weaker nucleophile. This makes it a great leaving group because it’s less likely to snatch back its electrons and prefers to leave the reaction zone.
In essence, the leaving group wants to be a “loner” and not re-attach to the molecule. This makes it a good leaving group, allowing the reaction to proceed. The stronger the base, the more likely it will be a bad leaving group.
What is the role of the leaving group in a nucleophilic reaction?
Let’s break down why this happens. A leaving group is an atom or a group of atoms that departs from a molecule during a reaction. It’s like a tag that gets removed from a piece of luggage. The ease with which a leaving group departs is determined by its stability. A stable leaving group is more likely to leave, because it can better handle the negative charge it carries away.
Think of it this way: a stable leaving group is like a strong swimmer who can confidently navigate the waters of the reaction, while a poor leaving group is like a hesitant swimmer who struggles to make it to the other side.
The formation of the carbocation is a key step in many nucleophilic reactions. A carbocation is a positively charged carbon atom that’s highly reactive. When a leaving group departs, it takes away an electron pair, leaving the carbon atom with a positive charge. This carbocation then attracts a nucleophile, which is an electron-rich species seeking a positive charge. The nucleophile attacks the carbocation, forming a new bond.
So, in essence, a good leaving group makes the carbocation formation fast, which allows the nucleophile to quickly attack the carbocation, resulting in a rapid reaction.
Here’s an analogy to illustrate this point: imagine you’re trying to put a puzzle together. The leaving group is like a piece of the puzzle that easily detaches from the rest. The faster the leaving group comes off, the faster you can find its matching piece and complete the puzzle. The nucleophile is like the matching piece that you’re trying to find, and the carbocation is the empty space in the puzzle where the matching piece fits. The faster the carbocation is formed, the faster you can find its matching piece and complete the puzzle.
What are the factors affecting the leaving group ability?
When a leaving group is more electronegative, it’s better at stabilizing the negative charge it develops when it departs from the molecule. This is because it can hold onto the electrons more tightly. A more stable leaving group is a better leaving group!
Think of it like this: imagine you’re trying to take a toy away from a child. If the child really loves the toy and clings to it tightly (like a less electronegative group), it’s going to be tough to pry it away. But if the child isn’t that interested in the toy and lets go easily (like a more electronegative group), it’s much easier to take it.
In the case of leaving groups, the stronger the negative charge the group can handle, the more readily it will depart from the molecule. This is because the leaving group is essentially taking the electrons with it. If it can stabilize this negative charge, it’s more likely to leave.
Here’s another way to think about it: a more electronegative leaving group is like a strong magnet – it’s better at holding onto the electrons and making the departure process more favorable. This makes it a more effective leaving group.
So, remember this key takeaway: electronegativity is a major factor in determining the effectiveness of a leaving group. The higher the electronegativity of the leaving group, the better it is at stabilizing the negative charge it acquires when it leaves the molecule, making it a more likely candidate to depart.
Why does nucleophilicity increase down the group?
You’re right! Negatively charged compounds are typically stronger nucleophiles than neutral ones. This is because they have an extra electron, making them more eager to donate it and form a bond.
Now, let’s focus on the trend down a group. As we move down a group, the electrons are less tightly held by the nucleus. This is because the electronegativity decreases. Think of it like this: the outer electrons are further away from the nucleus and experience less attraction.
This weaker hold on the electrons translates to a greater ability to donate them. The larger size of the atoms also plays a crucial role. These larger atoms have more electron clouds, creating a more diffuse negative charge. This makes them more reactive and increases their nucleophilicity.
Let me illustrate this with a simple analogy. Imagine a group of friends trying to hold onto a bouncy ball. The friends closer to the center hold on tightly, making it hard for the ball to escape. But the friends further away have a looser grip, allowing the ball to easily bounce out. The same principle applies to electrons. Those closer to the nucleus are held more tightly, while those further away are more readily available for sharing.
Therefore, when we move down a group, the increasing size and decreasing electronegativity of the atoms lead to a greater ability to donate electrons, resulting in stronger nucleophiles.
What is the leaving group ability proportional to?
Leaving group ability refers to how readily a group departs from a molecule during a chemical reaction. Think of it like a passenger getting off a bus – some passengers are quick and easy to disembark, while others take their sweet time. In chemistry, the ease with which a group leaves determines the reaction rate.
The leaving group ability is inversely proportional to the basicity of the leaving group. This means stronger bases are poorer leaving groups, while weaker bases are better leaving groups.
Here’s why:
Imagine a strong base like hydroxide ion (OH-). It’s very eager to grab a proton (H+) and form water. If it’s attached to a molecule, it’s more likely to stay put, holding onto the molecule rather than leaving as a separate ion.
Now consider a weak base like a halide ion (like chloride, Cl-). It has less of a desire for a proton. If attached to a molecule, it’s more willing to depart as a separate ion, leaving the molecule behind.
In essence, a good leaving group is a weak base that prefers to exist on its own rather than being part of a molecule. This concept is fundamental to understanding many organic reactions, especially those involving nucleophilic substitution and elimination.
Let’s dive deeper into this idea:
Think of the leaving group like a person on a swing set. If they’re very heavy (like a strong base), they’ll be reluctant to let go of the swing and leave it, even if they are given the opportunity. They’re “clingy,” preferring to stay attached to the swing (the molecule). But if they’re lighter (like a weak base), they’re more likely to release their grip and depart from the swing (the molecule).
This analogy highlights the key point: Leaving group ability is all about the leaving group’s preference to stay connected to the molecule or to move on independently. The stronger the base, the more it wants to hold onto the molecule, making it a poor leaving group. The weaker the base, the more it wants to be free, making it a good leaving group.
Is tosylate a better leaving group than iodide?
Let’s dive a little deeper into why this happens. The leaving group ability depends on several factors, including the stability of the leaving group after it departs. Tosylate, being a good leaving group, forms a stable anion due to its resonance stabilization. This makes it a great choice for many reactions.
However, the nature of the nucleophile can significantly influence the outcome. When we use ethoxide as a nucleophile, it’s a relatively strong base. This means it’s more likely to attack the carbon atom directly, leading to a straightforward SN2 reaction. In this scenario, tosylate, with its stable anion, readily departs.
On the other hand, thiolate is a stronger nucleophile and a better base than ethoxide. This allows it to react with tosylate to form a thioether, but it also makes it more likely to deprotonate the tosylate leaving group. When this happens, the tosylate anion loses its resonance stabilization and becomes less stable. This makes it a worse leaving group in this particular scenario.
Iodide and bromide, with their larger size and weaker base character, are less prone to deprotonation by the thiolate nucleophile. As a result, they remain stable leaving groups and are better choices in this situation.
In summary, the leaving group ability is not just a simple property of the leaving group itself, but is influenced by the nature of the nucleophile and the reaction conditions. This dynamic interplay is what makes organic chemistry so interesting and challenging!
What is directly proportional to nucleophilicity?
Let’s break down why this relationship exists. Nucleophilicity refers to a molecule’s ability to donate a pair of electrons to form a new bond. Basicity refers to a molecule’s ability to accept a proton (H+). Both concepts involve the availability of electrons for reaction.
Think of it this way: a strong base readily accepts a proton because it has a high electron density and a strong attraction for positive charges. This same high electron density makes it more likely to donate electrons and act as a nucleophile, attacking an electrophilic center (a region with a partial positive charge).
For example, consider the hydroxide ion (OH-). It’s a strong base because it readily accepts a proton, forming water (H2O). This same high electron density makes it an excellent nucleophile, readily attacking electrophiles.
However, it’s important to remember that nucleophilicity is not always directly proportional to basicity. There are several factors that can influence nucleophilicity besides basicity. These include:
Solvent: Polar protic solvents (like water or alcohols) can solvate (surround) nucleophiles, decreasing their reactivity. This effect is more pronounced for smaller, more highly charged nucleophiles.
Steric Hindrance: Bulky groups around the nucleophilic center can hinder its ability to attack an electrophile.
Leaving Group Ability: The ability of a group to leave a molecule after a nucleophilic attack can also affect the overall reaction rate.
So, while basicity is a good indicator of nucleophilicity, it’s not the only factor. To fully understand nucleophilicity, you need to consider all of these factors in their context.
Is the better the leaving group the faster the reaction?
The statement “the better leaving group leaves faster and thus the reaction can proceed faster” is spot on. This is the essence of what makes a good leaving group. A good leaving group is one that is able to stabilize the negative charge it acquires when it departs from the molecule.
Let’s break down how this applies to alcohol reactions. Under acidic conditions, the -OH group of an alcohol can be converted into a neutral water leaving group through protonation. This protonation makes the -OH a better leaving group because it turns it into a neutral water molecule, which is much more stable than the negatively charged hydroxide ion.
Here’s a simplified example to illustrate:
Imagine you have a group of friends, and one of them is really good at making new friends and being independent. They’re likely to leave the group more readily than someone who’s always clinging to the group and feeling lonely. This friend is like a better leaving group. They’re stable on their own, and they’re not going to hold back the group from doing other things.
The same principle applies in chemical reactions. A better leaving group is more stable on its own, and therefore leaves the molecule more readily. This allows the reaction to proceed faster, because the leaving group isn’t holding back the other reactants.
Factors that influence how good a leaving group is:
Stability of the leaving group: A more stable leaving group is a better leaving group. This is because the leaving group is more likely to accept the negative charge that it acquires when it departs from the molecule.
The ability of the leaving group to accommodate the negative charge: This is related to the stability of the leaving group. For example, a leaving group that can delocalize the negative charge through resonance or inductive effects will be a better leaving group.
The strength of the bond between the leaving group and the molecule: A weaker bond means the leaving group is easier to remove, which makes it a better leaving group.
In general, weak bases are better leaving groups because they are more stable on their own. This is because strong bases are highly reactive and are more likely to want to stay bonded to the molecule.
Let me know if you’d like to explore this further!
What determines a good leaving group?
Let’s break this down a bit more. Think of a leaving group like a passenger getting off a bus. A good leaving group is like a passenger who’s ready to go, doesn’t want to hang around, and doesn’t cause a fuss.
A weak base is like a passenger who’s ready to go, doesn’t want to hang around, and doesn’t cause a fuss. It doesn’t want to grab a proton (H+) and stay attached to the molecule.
A strong base is like a passenger who wants to stay on the bus and doesn’t want to get off. It’s more likely to grab a proton and stay attached to the molecule, making it a bad leaving group.
Here are some examples of good leaving groups:
Iodide (I-)
Bromide (Br-)
Chloride (Cl-)
Tosylate (TsO-)
Triflate (TfO-)
These groups are all weak bases, which makes them good leaving groups.
Let’s look at a few examples to help illustrate this.
Alkyl halides (like methyl chloride, CH3Cl) are good leaving groups because the halide ions (Cl-, Br-, I-) are weak bases.
Alcohols (like methanol, CH3OH) are poor leaving groups because the hydroxide ion (OH-) is a strong base. To make an alcohol a better leaving group, you can protonate it to form an oxonium ion (like CH3OH2+), which is a much weaker base.
Remember, the stability of the leaving group is important too. A good leaving group should be stable on its own, meaning it can exist independently after it leaves the molecule.
See more here: Is A Stronger Nucleophile A Better Leaving Group? | Nucleophilicity And Leaving Group Ability
What determines nucleophilicity and leaving-group ability?
So, what makes a nucleophile good? It’s all about how much it wants to share its electrons. A nucleophile is a species that loves to donate electrons. This electron-donating ability is all about the nucleophile’s energy and shape. The more readily available its electrons are, the more reactive it will be. It’s like having a big, fluffy cloud of electrons that’s eager to be shared.
Now, what makes a good leaving group? It’s the opposite! A leaving group is a species that wants to ditch the bond and go off on its own. A good leaving group is one that’s happy to depart, and that’s directly related to the strength of the bond it’s leaving. The weaker the bond, the easier it is for the leaving group to leave, and the more likely the reaction is to happen. Imagine a weak bond like a loose shoelace – it’s easy to untie!
Think of it this way: nucleophiles are like eager friends who want to share their stuff, and leaving groups are like people who are ready to go their separate ways. Both play important roles in chemical reactions.
Here’s an example. Let’s say we have a reaction involving a nucleophile attacking a molecule with a leaving group. A strong nucleophile will be more likely to attack and displace the leaving group if the leaving group has a weaker bond to the molecule. A weak leaving group, on the other hand, might not leave easily, even if a strong nucleophile is present.
Nucleophilicity and leaving group ability are very important concepts in organic chemistry. They help us understand how different molecules react and what kind of products we can expect. By understanding these concepts, we can design reactions to produce specific products or predict how a reaction will proceed.
Why does a good leaving group speed up a nucleophile reaction?
SN1 reactions are all about the leaving group, and that’s because the leaving group is directly involved in the rate-determining step. This means that how quickly the leaving group departs determines how fast the entire reaction goes.
Think of it this way: a good leaving group is like a friend who wants to go home early. They’re not attached to the party (the molecule) and are ready to leave quickly. The better a leaving group is, the faster it breaks the bond with the carbon atom, allowing the reaction to proceed.
Here’s a more detailed explanation:
A good leaving group is a stable molecule that can easily accommodate a negative charge. This means it’s more likely to leave on its own, creating a carbocation, which is a positively charged carbon atom. The formation of this carbocation is the rate-determining step of the reaction.
Once the carbocation is formed, the nucleophile can attack it. But, if the leaving group is a poor one, it’s stuck to the carbon atom, and the carbocation formation is slow. The nucleophile has to wait around, making the whole reaction slower.
So, how do you know if a leaving group is good or bad?
Good leaving groups are usually weak bases. Remember, a strong base loves to grab protons, so it’s not going to want to leave as a negatively charged species. Conversely, a weak base is more comfortable with a negative charge, so it will happily leave. Think of halides (like bromide, chloride, and iodide) or tosylate, they’re pretty good at being leaving groups.
Bad leaving groups are strong bases. They’re not happy leaving as a negatively charged species, so they make the reaction slow.
In summary, a good leaving group speeds up a nucleophilic reaction by making the rate-determining step, the formation of the carbocation, faster. The faster the leaving group departs, the faster the entire reaction goes.
Can a species be both a good nucleophile and a leaving group?
But here’s the catch! A good leaving group is also capable of breaking a bond and leaving the molecule. This is where the reaction conditions come in. The same species can be a good nucleophile in one reaction but a good leaving group in another. It all depends on the relative stability of the products formed.
For example, let’s consider the hydroxide ion (OH⁻). In a reaction where it’s reacting with a primary alkyl halide, the hydroxide ion will act as a good nucleophile, displacing the halide and forming an alcohol. This is because the resulting alcohol is more stable than the alkyl halide.
However, if we look at a different reaction, the hydroxide ion can also act as a good leaving group. For instance, in the reaction of an alcohol with a strong acid, the protonation of the alcohol makes the hydroxide ion a better leaving group, resulting in the formation of water. This is because water is a more stable molecule than the protonated alcohol.
So, the key takeaway is that a species can be both a good nucleophile and a good leaving group, depending on the specific reaction conditions and the relative stabilities of the products formed.
What are the trends in nucleophilicity?
Trends in Nucleophilicity
There are a few key trends to keep in mind:
Within a Period: As you move from left to right across a period, nucleophilicity generally decreases. This is because the electronegativity of the atoms increases, making them less likely to share their electrons.
Within a Group: As you move down a group, nucleophilicity generally increases. This is because the size of the atoms increases, which means their electrons are further away from the nucleus and more easily shared.
Charge: A negatively charged nucleophile is always more reactive than its neutral counterpart. This is because the negative charge makes it more attractive to the positively charged electrophile.
Steric Effects: The size and shape of a nucleophile can also affect its reactivity. Bulky nucleophiles are less reactive than smaller ones, because they have a harder time getting close to the electrophile.
Let’s break down each of these trends in more detail.
Within a Period: Imagine a row of chairs in a classroom, each representing an element in a period. The chairs on the left are the most willing to share, while the chairs on the right are holding on tight to their electrons. This is because elements on the left have lower electronegativity—they’re less greedy for electrons. For example, the nucleophilicity of OH- is greater than that of F- because oxygen is less electronegative than fluorine.
Within a Group: Now imagine a column of chairs, representing a group. As you move down the column, the chairs get bigger. This is because the elements at the bottom have more electron shells, making them larger and with electrons further away from the nucleus. Think of it as being easier to grab something from a big, open hand than a small, closed fist. The same principle applies to nucleophiles – the bigger they are, the more likely they are to share their electrons. For example, I- is more nucleophilic than F- because iodine is a larger atom.
Charge: Think of a negatively charged nucleophile as having a “magnet” attached to it. This magnet attracts the positively charged electrophile making it more likely to form a bond. A neutral nucleophile doesn’t have this extra attraction, so it’s less likely to react. For example, CH3O- is more nucleophilic than CH3OH because it carries a negative charge.
Steric Effects: Think of a big, bulky nucleophile as being clumsy. It might have a hard time getting close to the electrophile and making a good connection. This is because the bulky groups surrounding the reaction center create a hindrance, blocking the approach of the electrophile. For example, t-BuOK is less nucleophilic than CH3OK because the bulky t-butyl group hinders the approach of the electrophile.
These are just a few of the factors that affect nucleophilicity. It’s a complex topic, but hopefully, this explanation helps you get a better understanding of the trends involved!
See more new information: countrymusicstop.com
Nucleophilicity And Leaving Group Ability: A Powerful Duo In Organic Chemistry
Alright, so you’re diving into the world of organic chemistry, specifically nucleophilicity and leaving group ability, and you want to understand them like the back of your hand. Well, you’ve come to the right place! This is your comprehensive guide to getting a firm grasp on these fundamental concepts.
Let’s start by breaking down the basics. In a nutshell, nucleophilicity is a measure of how much a nucleophile wants to donate its electrons to form a new bond. Think of it as a measure of a molecule’s electron-donating power. On the other hand, leaving group ability is all about how easily a group can depart from a molecule, taking its electrons with it.
Nucleophilicity: The Electron Donators
Imagine nucleophiles as the electron-rich molecules in the chemical world. They’re always on the lookout for a positive charge or an electron-deficient atom to attack and share their electrons with. They’re like the generous party guests who always bring the snacks.
Think of these factors that influence nucleophilicity:
Charge: Negatively charged nucleophiles are generally more powerful because they have a surplus of electrons, making them more likely to donate them.
Electronegativity: The more electronegative an atom is, the more it holds onto its electrons, making it less likely to act as a nucleophile. So, the less electronegative, the better!
Steric Hindrance: Remember, atoms are not flat. They take up space. So, bulky nucleophiles with lots of atoms or groups around their reactive site can be hindered in their ability to attack. Think of them as a bulky, clumsy guest trying to squeeze through a crowded doorway – it takes more effort!
Solvent Effects: The solvent a reaction takes place in can have a significant impact on nucleophilicity. Protic solvents (like water or alcohol) can hydrogen bond with the nucleophile, reducing its reactivity. It’s like having a distracting conversation while trying to focus on something else. A polar aprotic solvent, like DMF or acetone, can be a better choice because they won’t hinder the nucleophile’s attack.
Leaving Group Ability: The Departing Guests
Now, let’s talk about leaving groups. They’re like the guests who’ve had their fill and are ready to leave the party. They’re stable molecules that can detach from the molecule they’re attached to, taking their electrons with them.
Think of these factors that influence leaving group ability:
Stability: A good leaving group is a stable molecule. It needs to be able to handle the extra electrons it takes with it when it leaves. The more stable it is, the easier it can detach from the molecule. Think of it like a strong friend who can handle the pressure of an awkward goodbye.
Electronegativity: A more electronegative atom will be a better leaving group because it can hold onto its electrons more tightly, making it easier to detach.
Size: Larger leaving groups are generally better because they can better accommodate the extra electrons they take with them. Think of it like a spacious suitcase that can hold everything you need to leave.
Understanding the Relationship
So, how do nucleophilicity and leaving group ability play together? They’re like two sides of the same coin. Nucleophiles attack a molecule, while leaving groups detach from it, leaving a new bond behind.
The better the leaving group, the easier it is for the reaction to occur. A strong nucleophile can attack a molecule even with a poor leaving group, but it will require more energy.
Practical Applications
These concepts are fundamental in organic chemistry. They are the foundation for understanding many essential reactions, like SN1 and SN2 reactions.
SN1 reactions are two-step reactions where the first step is the departure of the leaving group, forming a carbocation, and then the nucleophile attacks the carbocation. In SN2 reactions, the nucleophile directly attacks the molecule, displacing the leaving group in a single step.
FAQs
1. What are some common examples of nucleophiles?
Common examples of nucleophiles include:
Hydroxide ions (OH-)
Alkoxide ions (RO-)
Ammonia (NH3)
Amines (RNH2)
Water (H2O)
Halides (Cl-, Br-, I-)
2. What are some common examples of leaving groups?
Common examples of leaving groups include:
Halides (Cl-, Br-, I-)
Tosylates (OTs)
Mesylates (OMs)
Triflates (OTf)
Water (H2O)
3. How can I predict if a reaction will occur based on nucleophilicity and leaving group ability?
A good rule of thumb is that a reaction is more likely to occur if the nucleophile is strong and the leaving group is good. However, you should also consider the reaction conditions and the specific molecules involved.
4. What is the difference between nucleophilicity and basicity?
While both nucleophilicity and basicity relate to electron donation, they are not the same thing. Basicity refers to the ability of a molecule to donate electrons to a proton (H+). Nucleophilicity is a broader term that refers to the ability of a molecule to donate electrons to any electrophile.
5. What are some examples of reactions where nucleophilicity and leaving group ability are important?
Nucleophilicity and leaving group ability are critical in many organic reactions, including:
Substitution reactions (SN1 and SN2)
Addition reactions
Elimination reactions
Ring-opening reactions
6. How can I improve my understanding of nucleophilicity and leaving group ability?
Practice, practice, practice! Work through problems and examples to reinforce your understanding.
Study the mechanisms of various reactions. Understanding the step-by-step process will give you insight into the role of nucleophiles and leaving groups.
Consult textbooks and online resources. There are plenty of resources available to deepen your knowledge.
Ask questions! Don’t hesitate to reach out to your instructor or classmates for help.
There you have it, a deep dive into nucleophilicity and leaving group ability! Remember, understanding these concepts is key to mastering organic chemistry. You’re well on your way to becoming an expert in the field. Keep practicing, stay curious, and you’ll be a pro in no time!
Nucleophilicity and Leaving-Group Ability in
A clear picture emerges from these analyses: nucleophilicity is determined by the electron-donor capability of the nucleophile (i.e., energy and shape of the X − np atomic orbital), and ACS Publications
Leaving Groups – Chemistry LibreTexts
Resonance Increases the Ability of the Leaving Group to Leave: As we learned previously, resonance stabilized structures are weak bases. Therefore, leaving Chemistry LibreTexts
Nucleophile – Chemistry LibreTexts
Now that we have determined what will make a good leaving group, we will now consider nucleophilicity. That is, the relative strength of the nucleophile. Chemistry LibreTexts
What makes a good leaving group? – Master Organic Chemistry
A leaving group is a nucleophile acting in reverse; it accepts a lone pair as the bond between it and its neighbor (usually carbon for our purposes) is broken. So Master Organic Chemistry
Effects of Solvent, Leaving Group, and Nucleophile on
Effects of Leaving Group. An S N 1 reaction speeds up with a good leaving group. This is because the leaving group is involved in the rate-determining step. A good Chemistry LibreTexts
A Unified Framework for Understanding Nucleophilicity and …
According to the IUPAC Gold Book, nucleophilicity is the relative reactivity of nucleophilic reagent and the leaving-group ability (nucleofugality) is the tendency of National Center for Biotechnology Information
Influence of the leaving group on the dynamics of a gas
In addition to the nucleophile and solvent, the leaving group has a significant influence on S N 2 nucleophilic substitution reactions. Its role is frequently discussed Nature
Leaving group – Wikipedia
In chemistry, a leaving group is defined by the IUPAC as an atom or group of atoms that detaches from the main or residual part of a substrate during a reaction or elementary Wikipedia
Nucleophilicity – an overview | ScienceDirect Topics
Nucleophilicity refers to the ability of a nucleophile to displace a leaving group in a substitution reaction. We describe various trends in nucleophilicity in this section. ScienceDirect
Nucleophilic Substitution (SN2): Dependence on
They found that the nucleophilicity is determined by the electron-donor capability of the nucleophile (energy and shape of the X − np atomic orbital), and the leaving-group ability is derived directly Chemistry Europe
Nucleophiles, Electrophiles, Leaving Groups, And The Sn2 Reaction
Nucleophilic Strength
Leaving Group Stability – Sn1 And Sn2 Reactions
Nucleophilicity Comparison Trick Organic Chemistry | Iit Jee \U0026 Neet | Vineet Khatri | Atp Star Kota
Basicity Vs Nucleophilicity – Steric Hindrance
Link to this article: nucleophilicity and leaving group ability.
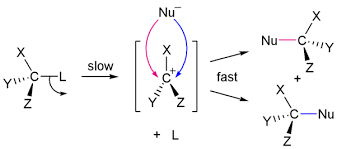
See more articles in the same category here: blog https://countrymusicstop.com/wiki